Fat Saturation/ Fat Suppression Techniques
Fat saturation, also known as fat suppression, is a technique used to suppress the signal from fat tissues in order to enhance the visibility of other tissues or structures of interest. Fat saturation is particularly useful in areas where fat can interfere with the interpretation of the MRI images.
The basic principle behind fat saturation is that fat has a different resonance frequency compared to other tissues in the body. By applying a radiofrequency pulse at the resonance frequency of fat, the fat signal can be selectively suppressed while leaving the signals from other tissues relatively unaffected.
The fat saturation technique typically involves three main steps:
Pre-saturation: Before acquiring the actual MRI images, a radiofrequency pulse is applied to selectively excite the fat tissue. This causes the fat protons to precess, and the signal is phase-shifted.
Time delay: After the pre-saturation pulse, there is a time delay known as the “fat saturation time.” During this delay, the fat signal decays while other tissue signals remain relatively constant.
Image acquisition: The MRI images are acquired after the fat saturation time, during which the fat signal has decayed. Since the fat signal is suppressed, the resulting images have reduced or eliminated fat signal, thereby improving the visibility of other tissues or structures.
Fat saturation can be achieved using different techniques. The main types of fat saturation techniques used in MRI are:
- Chemical Shift Selective (CHESS) fat saturation
- Spectral Adiabatic Inversion Recovery (SPAIR) fat saturation
- Short Tau Inversion Recovery (STIR)
- Water excitation fat saturation
- Dixon
Chemical Shift Selective (CHESS)
Chemical fat saturation is a technique used in MRI (Magnetic Resonance Imaging) to selectively suppress the signal from fat protons while preserving the signal from other tissues. It relies on the inherent difference in the resonant frequencies between water and fat protons.
In this technique, a selective RF (Radiofrequency) pulse is applied that specifically targets the fat resonance frequency. This pulse effectively saturates the fat spins by flipping their magnetization, reducing their signal intensity. Meanwhile, the water protons, having a different resonant frequency, are minimally affected by the RF pulse and retain their magnetization.
Chemical Shift Selective (CHESS) fat saturation images
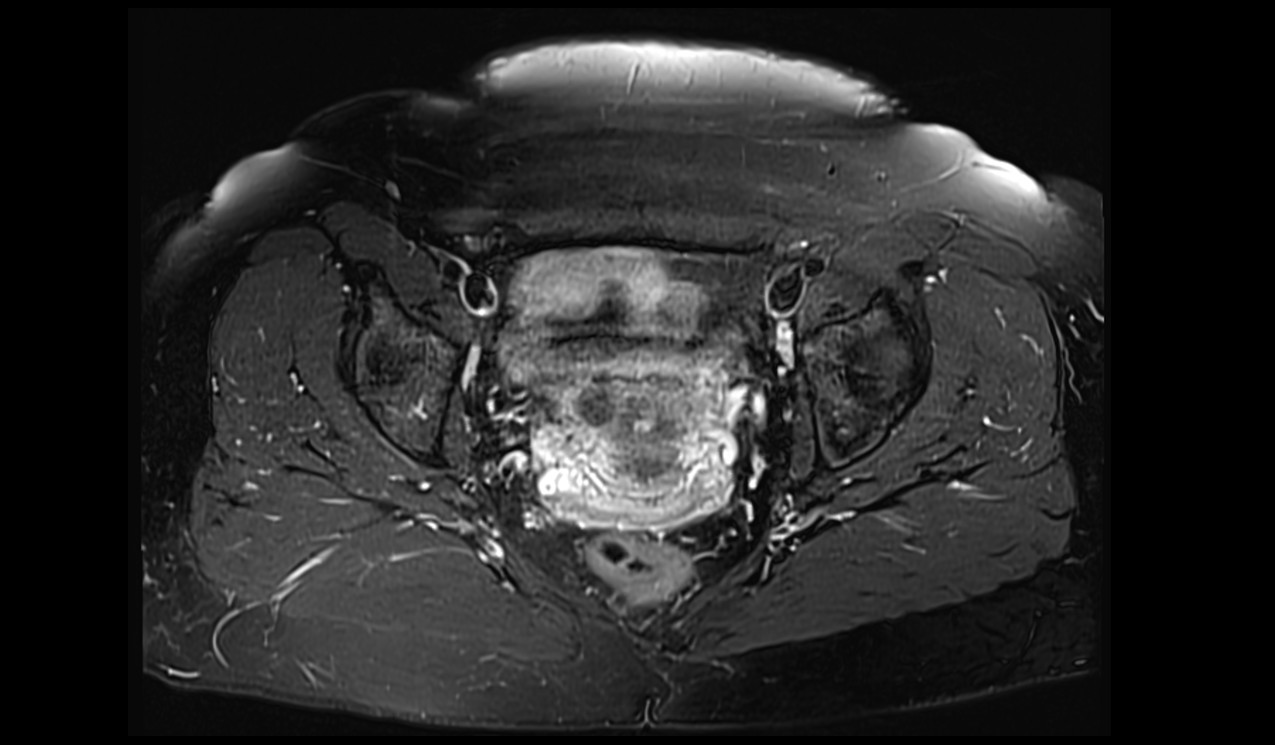
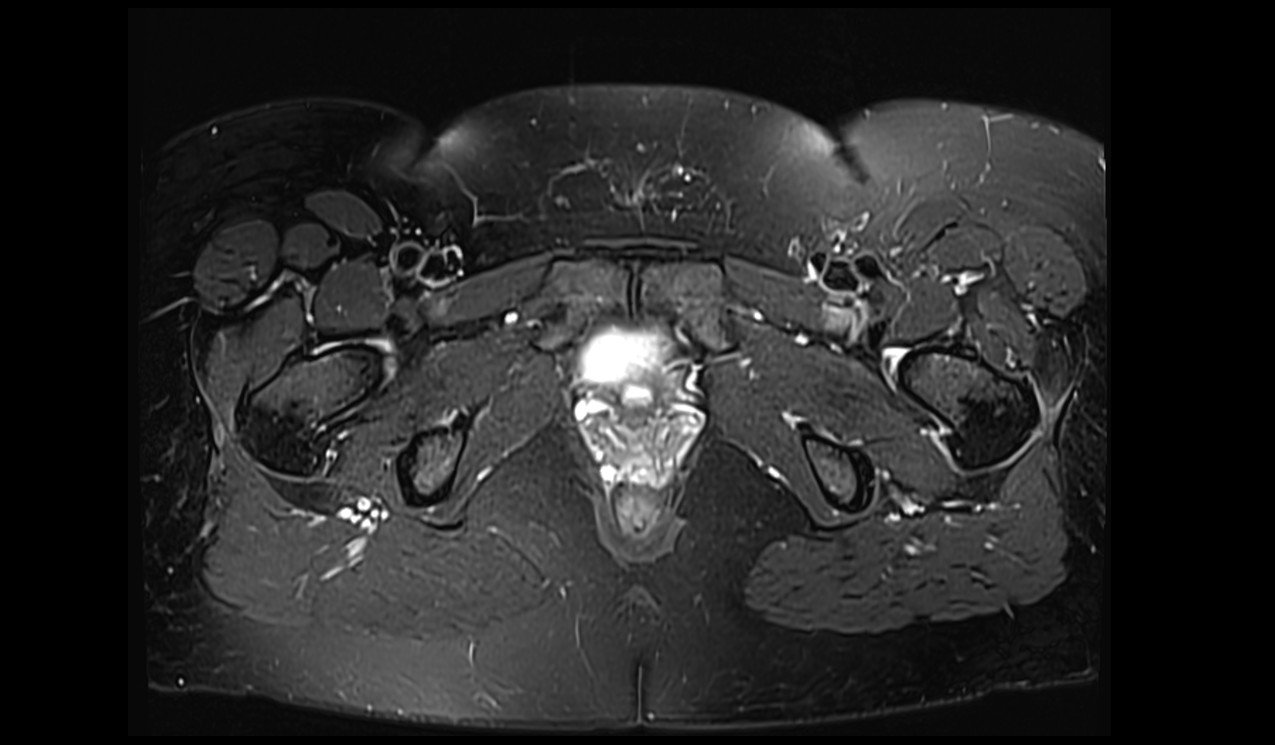
Advantages of chemical fat saturation:
Effective fat suppression: The technique allows for significant reduction or elimination of fat signal, enhancing the visibility of other tissues or structures of interest.
Improved tissue characterization: By selectively suppressing the fat signal, chemical fat saturation enables better differentiation between fat and other tissues, leading to improved tissue characterization and diagnostic accuracy.
Compatibility with various imaging sequences: Chemical fat saturation can be utilized in different imaging sequences, such as T1-weighted or T2-weighted sequences, allowing flexibility in image acquisition.
Disadvantages of chemical fat saturation:
Sensitivity to magnetic field inhomogeneities: Variations in the magnetic field across different regions of the body or within patients can result in incomplete fat saturation. This can lead to residual fat signal or inconsistent fat suppression.
Variations in fat composition: Different types of fat have slightly different resonant frequencies. This can affect the accuracy and reliability of fat saturation, as the RF pulse may not perfectly match the resonant frequency of all fat protons.
Potential for artifacts: Motion artifacts, such as patient movement or respiratory motion, can affect the effectiveness of fat suppression and introduce image artifacts.
Spectral Adiabatic Inversion Recovery (SPAIR)
SPAIR (Spectral Adiabatic Inversion Recovery) is a fat saturation technique used in MRI (Magnetic Resonance Imaging) to selectively suppress the signal from fat protons while preserving the signal from other tissues. SPAIR employs a spectral adiabatic inversion pulse to achieve fat saturation.
The SPAIR fat saturation technique works by applying a tailored RF (Radiofrequency) pulse that selectively inverts the magnetization of fat protons. This inversion pulse is designed to cover a range of frequencies corresponding to the resonance frequency of fat. As a result, the fat protons’ magnetization is flipped, effectively suppressing their signal during the subsequent image acquisition. In contrast, the magnetization of other tissues, such as water protons, remains unaffected and retains its signal.
Spectral Adiabatic Inversion Recovery (SPAIR) fat saturation images
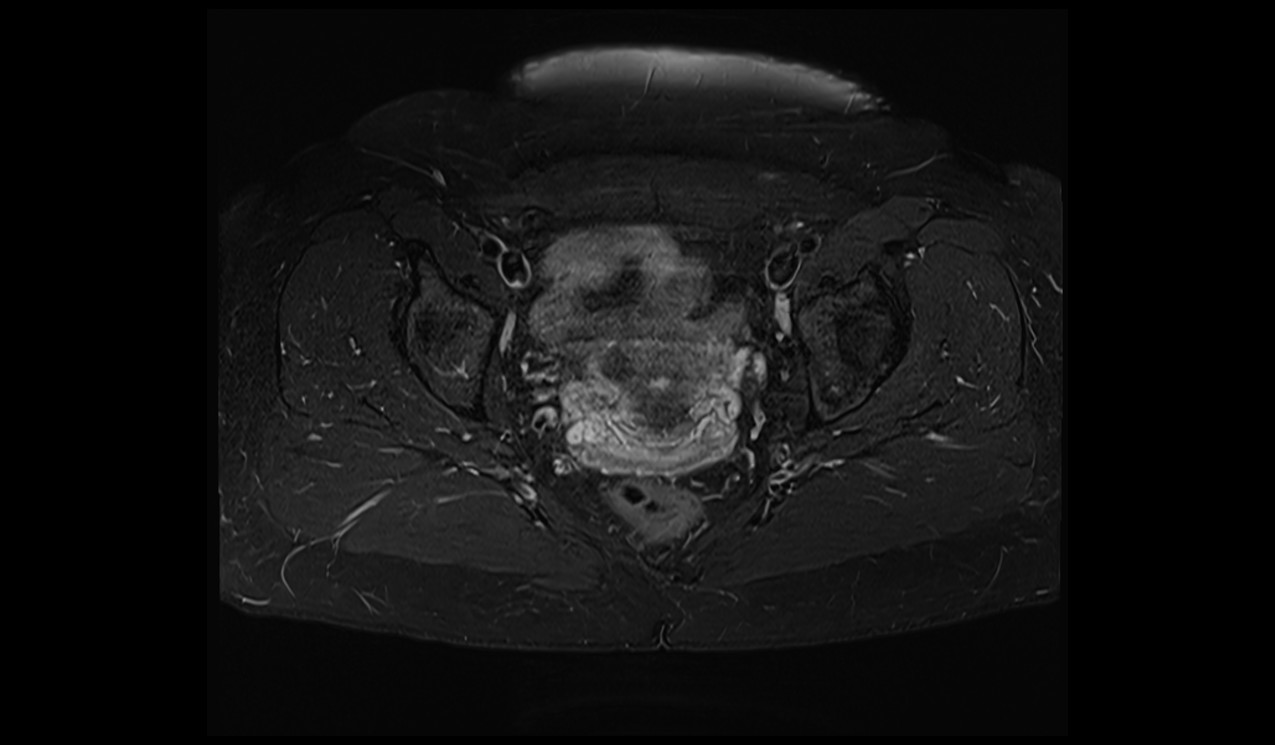
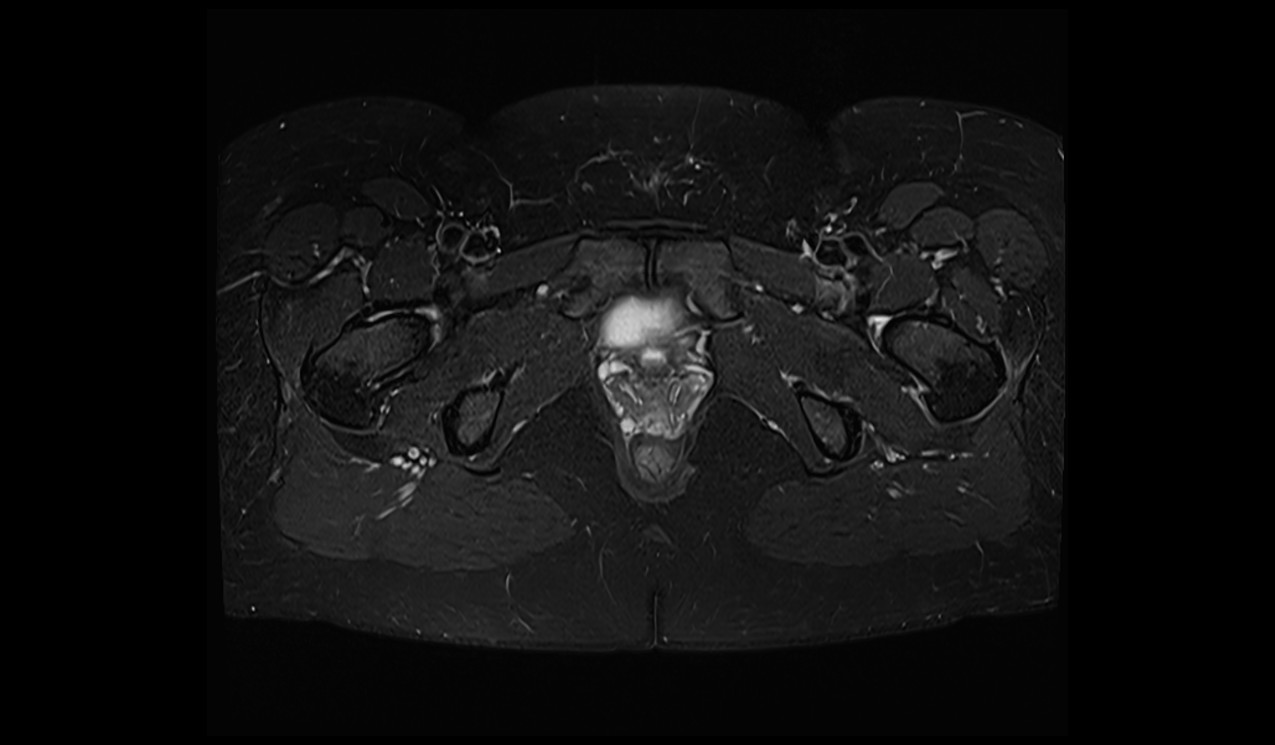
Advantages of the SPAIR:
Effective fat suppression: SPAIR provides robust fat suppression, reducing or eliminating the signal from fat protons. This improves image contrast and enables better visualization of other tissues or structures of interest.
Compatibility with various imaging sequences: SPAIR can be applied in different imaging sequences, including both T1-weighted and T2-weighted sequences, providing flexibility in image acquisition.
Versatility across different body regions: SPAIR is effective in various body regions, including regions with a higher fat content. It can be applied to different anatomical regions, enabling consistent fat saturation throughout the body.
Disadvantages of the SPAIR:
Sensitivity to magnetic field inhomogeneities: In regions with magnetic field variations, such as near air-tissue interfaces or metal implants, the effectiveness of SPAIR may be compromised, resulting in incomplete fat suppression.
Potential for incomplete fat saturation: Depending on the specific imaging parameters and patient characteristics, SPAIR may not achieve complete fat suppression in every case. Residual fat signal may still be present, reducing the effectiveness of fat suppression.
Increased scan time: The application of additional RF pulses for fat saturation can slightly increase the overall scan time, although the impact is generally minimal.
Short Tau Inversion Recovery (STIR)
STIR (Short Tau Inversion Recovery) is a fat saturation technique used in MRI (Magnetic Resonance Imaging) to selectively null the signal from fat protons, thereby improving image contrast and visualization of other tissues. STIR is based on the principle of inversion recovery, where a non-selective inversion pulse with a short inversion time (TI) is applied.
In the STIR technique, the TI is set to match the T1 relaxation time of fat, which is typically around 150-200 milliseconds. When the inversion pulse is applied, it inverts the magnetization of fat protons, making them temporarily aligned in the opposite direction to the external magnetic field. Subsequently, during the image acquisition, the fat signal is suppressed, while other tissues retain their signal. This suppression of fat signal enhances the visibility of structures and pathologies in the body.
Short Tau Inversion Recovery (STIR) images
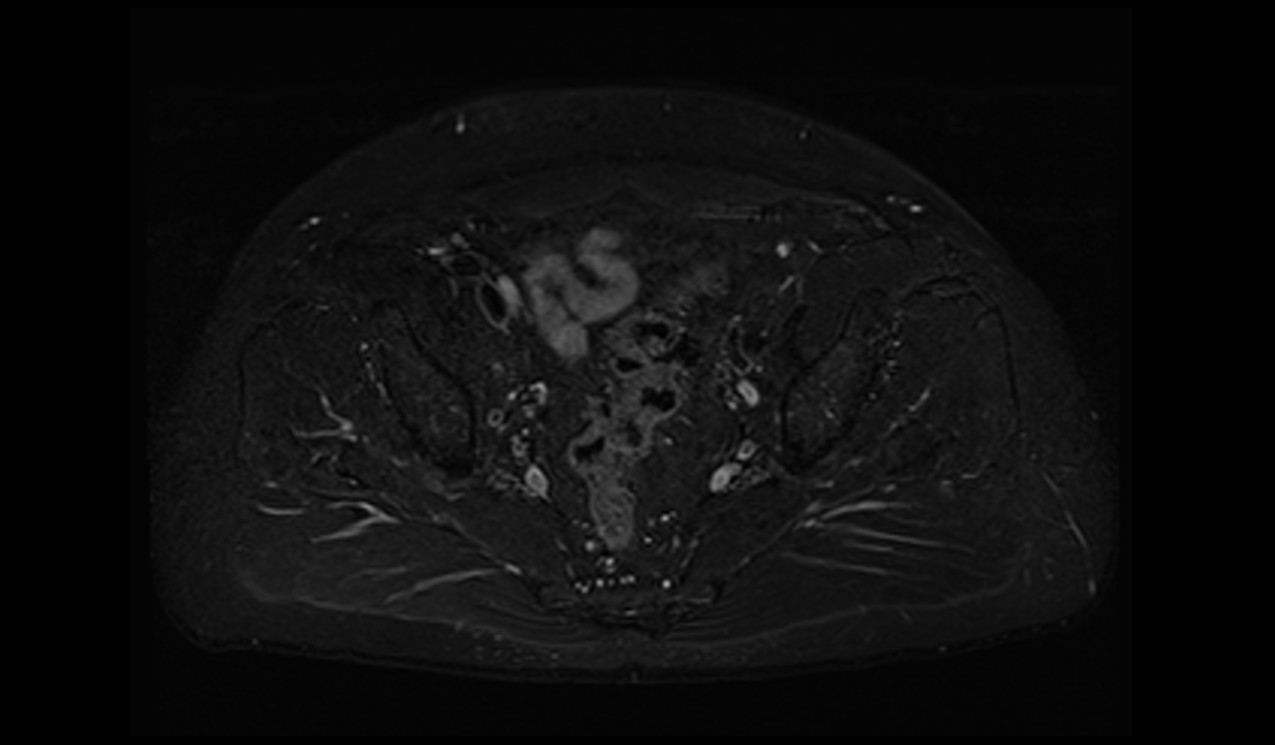
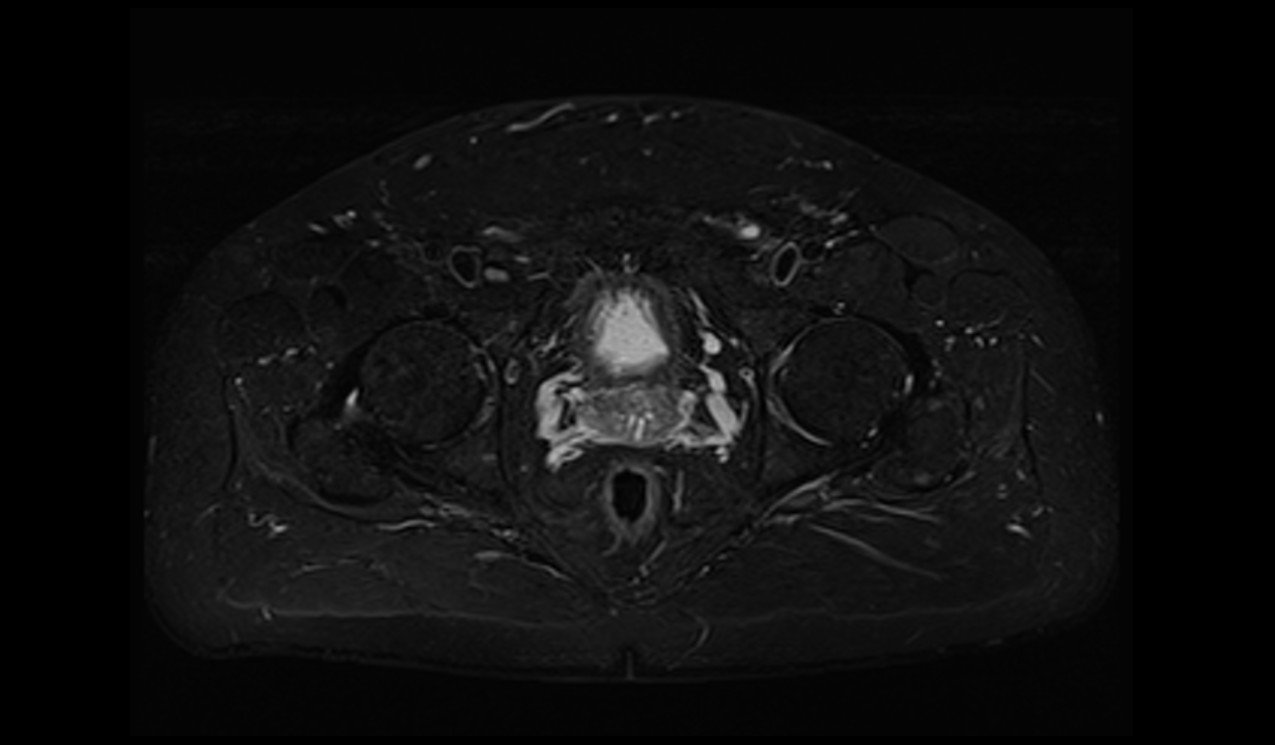
Advantages of the STIR fat saturation:
Uniform fat suppression: STIR provides relatively uniform fat suppression across different regions of the body. It helps ensure consistent fat saturation, leading to improved image quality and diagnostic accuracy.
Improved tissue contrast: By suppressing the signal from fat, STIR enhances the contrast between fat and other tissues, making it easier to distinguish abnormalities or structures of interest.
Disadvantages of the STIR fat saturation:
Sensitivity to the choice of inversion time (TI): The effectiveness of STIR depends on selecting an appropriate TI that matches the T1 relaxation time of fat. If the TI is not accurately set, incomplete fat suppression or unintended suppression of other tissues may occur.
Sensitivity to magnetic field inhomogeneities: Variations in the magnetic field across different body regions or within patients can affect the accuracy of fat saturation, potentially leading to incomplete fat suppression.
Water Excitation Fat Saturation
Water excitation is a fat saturation technique used in MRI (Magnetic Resonance Imaging) to selectively excite the water signal while suppressing the signal from fat protons. This technique takes advantage of the different resonant frequencies of water and fat to enhance image contrast and improve visualization of structures.
In water excitation, specific RF (Radiofrequency) pulses or pulse sequences are employed to target the resonant frequency of water protons. These RF pulses are designed to selectively excite the water signal, while attenuating or nulling the signal from fat protons. As a result, the water signal becomes prominent in the acquired images, while the contribution from fat is reduced or eliminated.
Advantages of the water excitation fat saturation:
Effective fat suppression: Water excitation offers robust fat suppression by selectively exciting the water signal and attenuating the signal from fat. This improves image contrast and enables better visualization of other tissues or structures of interest.
Enhanced tissue characterization: By selectively exciting water and minimizing the signal from fat, water excitation improves the ability to differentiate between different tissues, aiding in tissue characterization and diagnostic accuracy.
Improved vessel visualization: Water excitation is particularly useful in angiography and vascular imaging, as it can enhance the visualization of blood vessels by reducing the signal from surrounding fat tissues.
Disadvantages water excitation fat saturation:
Sensitivity to magnetic field inhomogeneities: Variations in the magnetic field strength across different regions of the body or within patients can impact the effectiveness of water excitation, potentially leading to incomplete fat saturation.
Variations in fat composition: Different types of fat have slightly different resonant frequencies. This can affect the accuracy of water excitation, as the RF pulses may not precisely match the resonant frequency of all fat protons, resulting in incomplete fat suppression.
The Dixon fat saturation technique is a method used in MRI (Magnetic Resonance Imaging) to separate the signals from fat and water protons, allowing for fat suppression and improved tissue characterization. It is based on exploiting the chemical shift difference between fat and water.
DIXON
Dixon fat saturation in MRI is a technique based on the chemical shift difference between fat and water protons. During Dixon imaging, multiple images with different echo times (TEs) are acquired. The phase shifts caused by the chemical shift difference between fat and water protons are captured in these images. By analyzing these phase shifts, the Dixon algorithm can separate the fat and water components from the acquired data.
The Dixon algorithm uses mathematical calculations to estimate the fat and water signal contributions for each pixel or voxel in the image. This separation process results in the generation of separate fat-only and water-only images, where the fat signal is suppressed in the water-only images.
Dixon fat saturation images
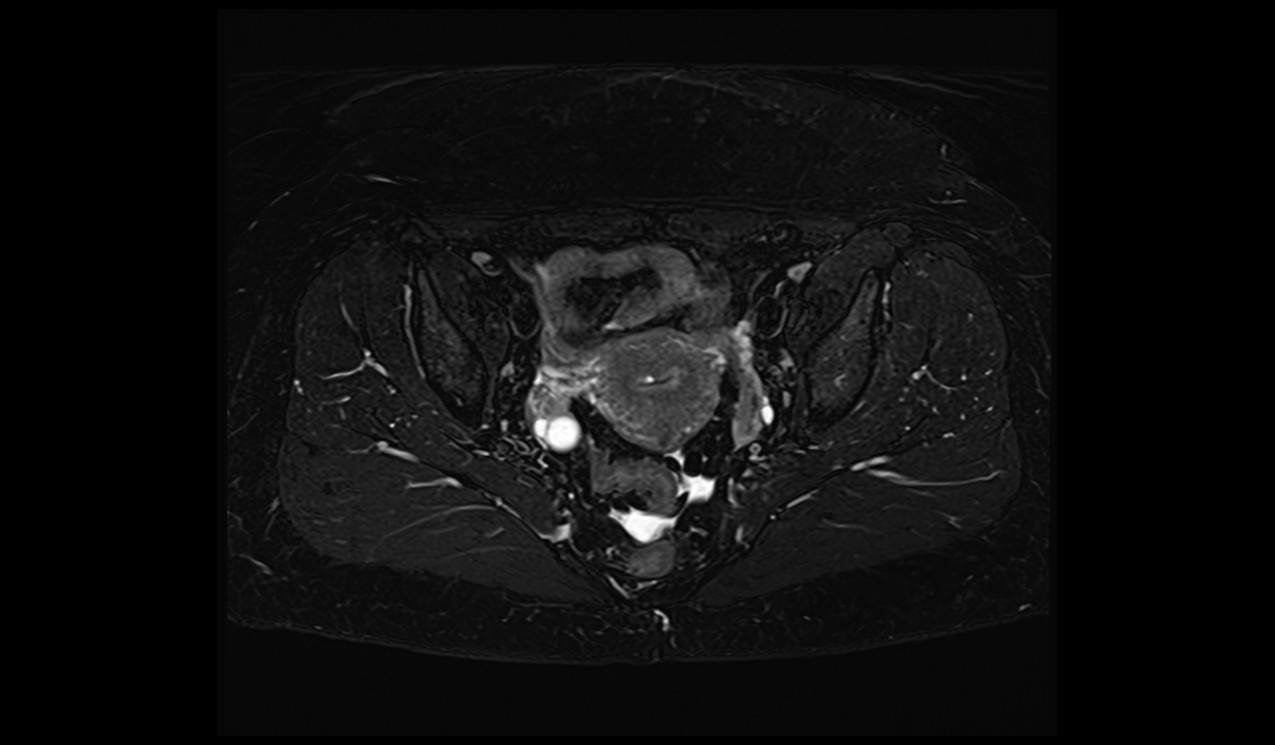
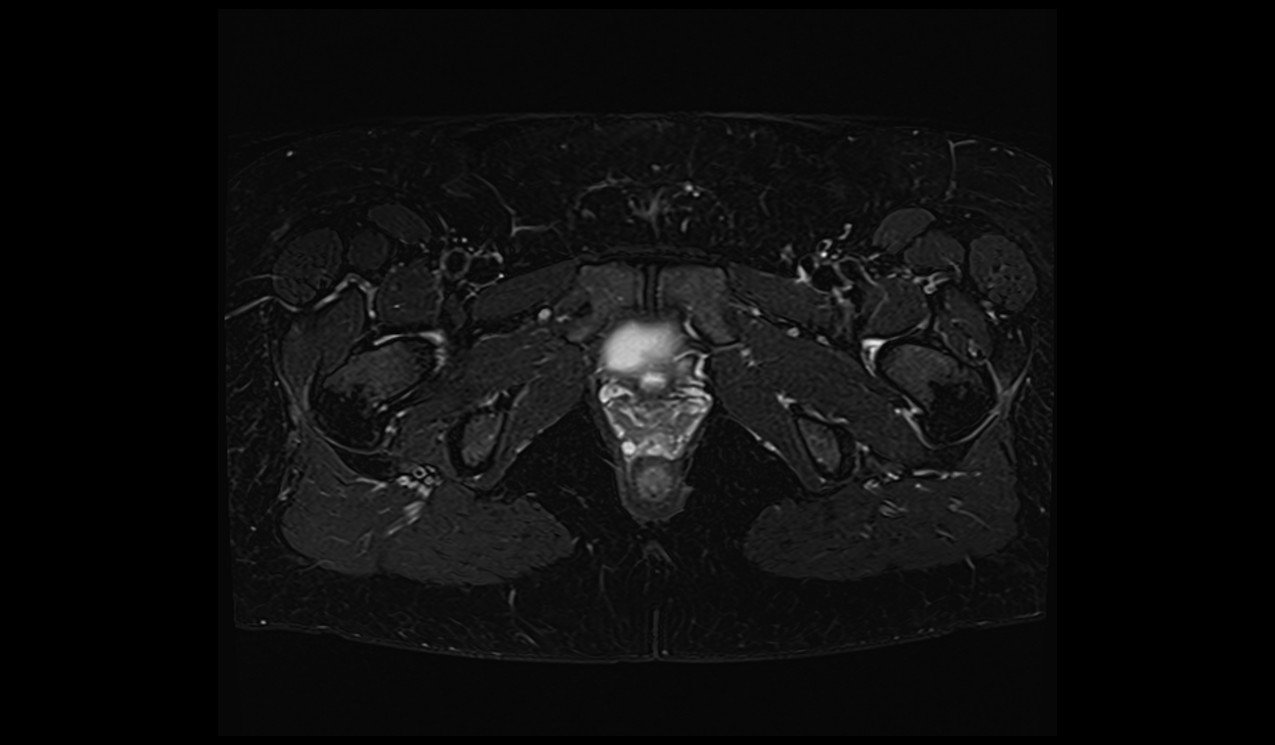
Advantages of the Dixon fat saturation:
Effective fat suppression: The Dixon technique enables robust fat suppression, as the fat-only images can be generated by separating the fat signal from the acquired data. This enhances image contrast and improves visualization of other tissues or structures.
Improved tissue characterization: By generating separate fat-only and water-only images, the Dixon technique provides better tissue characterization. It allows for the differentiation between fat, water, and other tissues, leading to improved diagnostic accuracy.
Quantitative fat fraction mapping: The Dixon technique can provide quantitative fat fraction maps, which quantify the proportion of fat in tissues. This is useful for assessing fat content and monitoring conditions such as fatty infiltration or steatosis.
Disadvantages of the Dixon fat saturation:
Sensitivity to motion artifacts: The Dixon technique can be sensitive to motion artifacts, such as patient movement or respiratory motion, as these can introduce phase inconsistencies in the acquired data. Motion correction techniques are often employed to mitigate these artifacts.
Susceptibility to magnetic field inhomogeneities: Variations in the magnetic field across different regions of the body or within patients can affect the accuracy and reliability of the Dixon technique, leading to inconsistencies in fat-water separation and potential artifacts.
Increased scan time: The acquisition of multiple images with different echo times extends the overall scan time, which can be a limitation in time-sensitive or patient-dependent imaging scenarios.
References
- Dixon, W. T. (1984). Simple proton spectroscopic imaging. Radiology, 153(1), 189-194.
- Glover, G. H. (1991). Multipoint Dixon technique for water and fat proton and susceptibility imaging. Journal of Magnetic Resonance Imaging, 1(5), 521-530.
- Reeder, S. B., Wen, Z., Yu, H., Pineda, A. R., Gold, G. E., Markl, M., … & Brittain, J. H. (2004). Multicoil Dixon chemical species separation with an iterative least-squares estimation method. Magnetic Resonance in Medicine, 51(1), 35-45.
- Bydder, M., & Yokoo, T. (2017). Imaging and fat quantification. In Quantitative MRI of the Liver (pp. 11-26). Springer.
- Karampinos, D. C., Yu, H., Shimakawa, A., Link, T. M., Majumdar, S., & Norbeck, O. C. (2011). T1-corrected fat quantification using chemical shift-based water/fat separation: application to skeletal muscle. Magnetic Resonance in Medicine, 66(5), 1312-1326.
- Dixon, A. K. (2014). Techniques for imaging fat. Clinical Radiology, 69(8), 839-845
- Meijer, F.J., et al. (1992). SPIR fat suppression in MR imaging: clinical experience. Radiographics, 12(2), 289-296.
- De Bondt, P., et al. (2003). Short tau inversion recovery (STIR) imaging: utility in musculoskeletal MRI. European Radiology, 13(10), 2131-2137.
- Glover, G.H. (1991). Multipoint Dixon technique for water and fat proton and susceptibility imaging. Journal of Magnetic Resonance Imaging, 1(5), 521-530.
- Pattany, P.M., et al. (1993). Fast spin-echo MR imaging: clinical applications for neuroimaging. Radiographics, 13(6), 1327-1342.