Number Of Excitations (NEX/NSA) and Image Quality
Introduction
Number of excitations (NEX) or number of signal averages/acquisitions (NSA) are measurement parameters. They are used to represent the number of times each line of k-space data is acquired and are primarily used to improve the signal-to-noise (SNR) ratio. Doubling the NEX only improves the SNR by the square root of two (√2) because random noise is also sampled. The noise in the MRI image is from electromagnetic noise in the body resulting from molecular movement and electrical resistance of the receiver coils. Therefore, as the number of NEX increases, noise begins to be cancelled out. Repeated sampling and the accumulation of signal result in a high-signal image.
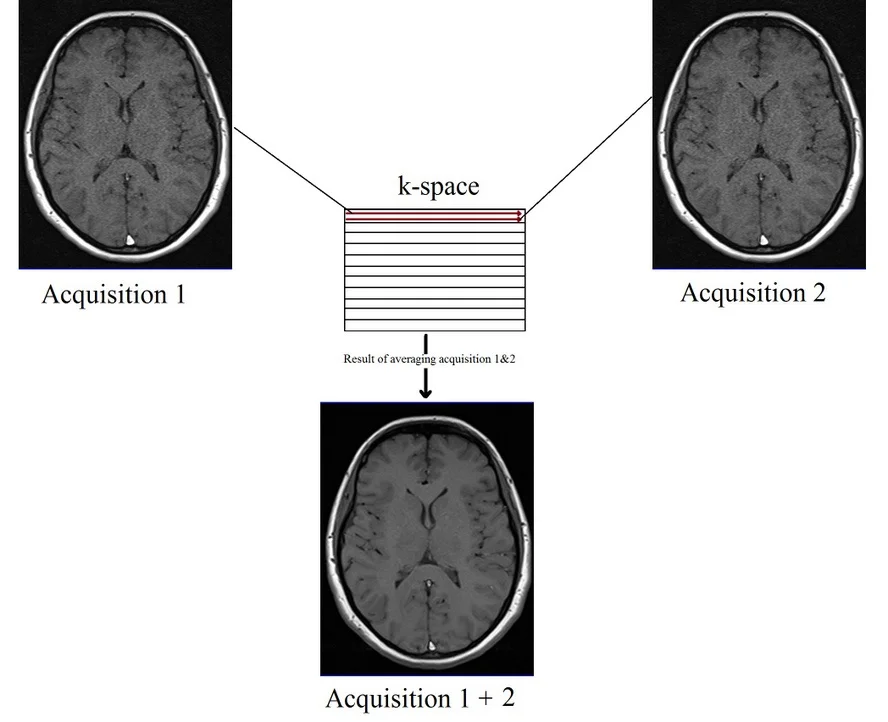
Doubling the NEX will increase the SNR by 140% and double the scan time. For example, if you take a sequence with a scan time of 2 minutes, NEX of 1, and SNR of 1 (100%), increasing the NEX from 1 to 2 will extend the scan time to 4 minutes and the SNR by 140%, or 1.4.
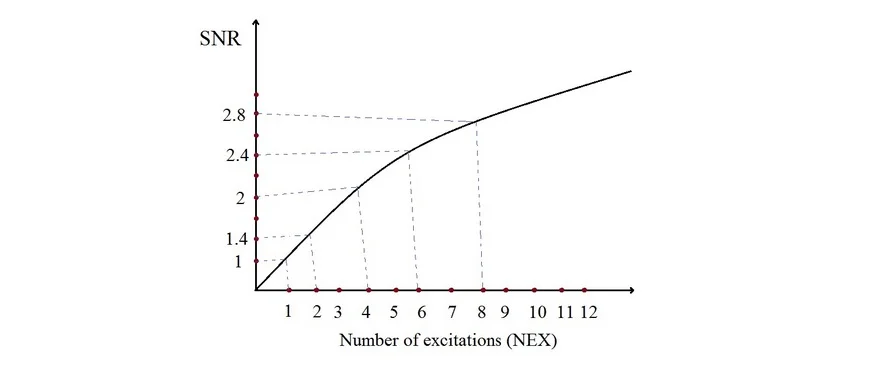
Practical applications of Number of excitations (NEX\NSA)
NEX can be used to increase SNR in high-resolution imaging with small fields of view. Employing multiple signal excitations is also effective in reducing motion and pulsation artifacts. The only disadvantage of increasing NEX is the significant increase in acquisition time.
Number of Excitations (NEX) and Slice Thickness
Decreasing the slice thickness in high resolution imaging will reduce the SNR. The most appropriate way of increasing the SNR is to increase NEX. For example, if a T1 TSE sequence with a slice thickness of 4mm, NEX 1, SNR 1 and acquisition time of 2 minutes is changed to a slice thickness of 2mm, the signal will drop to 0.49 or 49%. In order to compensate for the signal drop, the user needs to increase the NEX from 1 to 3 but will increase the scan time from 2 to 6 minutes. This is the most appropriate method when reducing the slice thickness without compromising image quality.
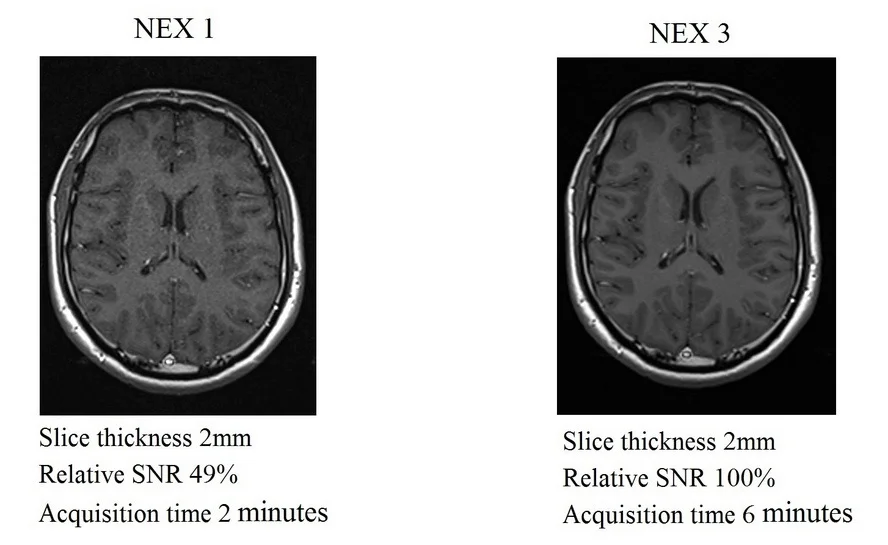
Number of Excitations (NEX) and Field of View (FOV)
Decreasing the field of view (FOV) will reduce the pixel size and result in an image with low signal-to-noise ratio (SNR). To compensate for signal loss, the user must either increase the number of excitations (NEX) or reduce the matrix size. Decreasing the matrix size will slightly lower the image quality, while increasing the NEX will enhance image quality. However, the main drawback of increasing NEX is a significant increase in scan time.
For instance, if a T1-weighted turbo spin echo (TSE) sequence with a 200mm FOV, NEX of 1, SNR of 1, and an acquisition time of 2 minutes is adjusted to a 100mm FOV, the signal will decrease to 0.39 or 39% of the original. In this case, the user should raise the NEX from 1 to 4, extending the scan time from 2 minutes to 8 minutes. This approach is the most suitable for achieving high-resolution imaging with a very small FOV, such as temporomandibular joint (TMJ) and pituitary imaging.
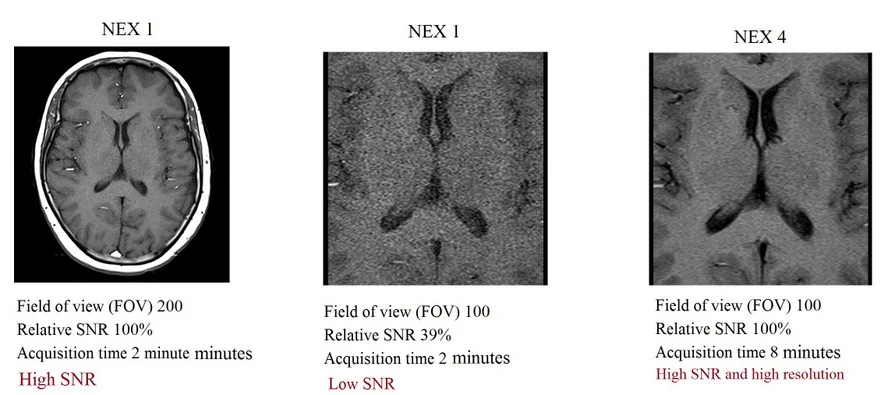
The same principle can be used to reduce scan time when increasing the FOV, as increasing the FOV results in an increase in the SNR. In order to mitigate the surplus signal, the user can lower the NEX. For instance, considering a T1 TSE sequence with an acquisition time of 6 minutes, a 100mm FOV, NEX set at 2, and SNR at 1, enlarging the FOV from 100mm to 200mm will double the signal, resulting in a 200% increase. To counteract the excess signal, the user can decrease the NEX from 2 to 1, effectively reducing the scan time from 6 minutes to 3 minutes.
Number of Excitations (NEX) and Resolution
Increasing the matrix size will reduce the pixel size and result in a low SNR image. To compensate for signal loss, the user can increase the NEX. However, increasing either the matrix size or NEX will lead to an increase in scan time. If both of these parameters are increased, the scan time will nearly triple. Despite this, the outcome will be a sharp and highly detailed image. For instance, consider a T1 TSE sequence with the following parameters: a 256×256 matrix, NEX 1, SNR 1, and an acquisition time of 2 minutes.
When the matrix size is increased from 256×256 to 320×320, the SNR drops to 0.72 (or 72%). Simultaneously, the scan time extends to 2.31 minutes. To counteract the signal drop, the user needs to raise the NEX from 1 to 2, which, in turn, increases the scan time from 2.31 to 5.02 minutes. Nonetheless, this method remains the most appropriate choice for performing high-resolution pelvic imaging, where both sharp detail and high SNR are essential.
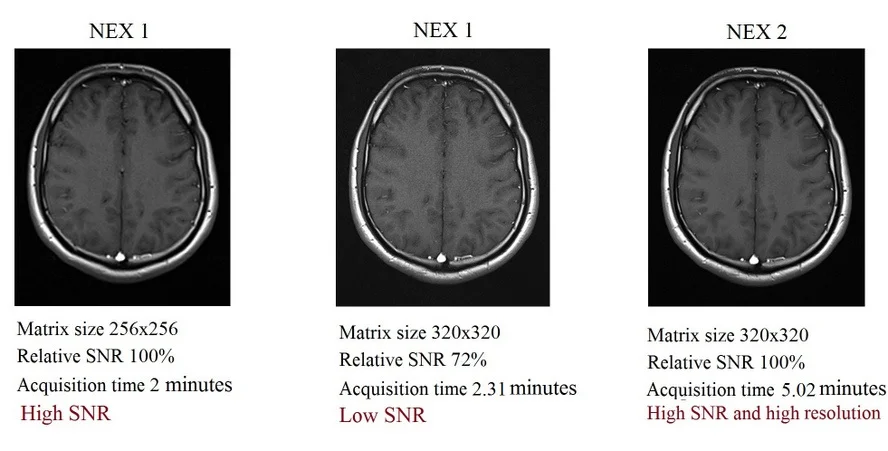
Number of Excitations (NEX) and Bandwidth
Increasing the receiver bandwidth will reduce SNR, and increasing NEX is the most appropriate technique to address this issue. For instance, consider a T1 TSE sequence with a receiver bandwidth of 100 Hz, NEX of 2, and a relative SNR of 1. If the bandwidth is increased from 100 to 250 Hz, the new SNR will decrease to 0.49. Compensating for this signal loss involves raising the NEX from 2 to 3. However, it’s important to note that this adjustment will significantly extend the scan time.
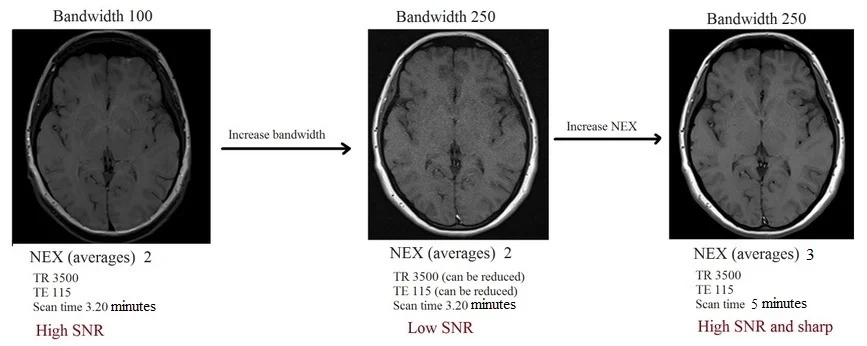
Number of Excitations (NEX) and Artefacts
Motion artefact is a form of noise commonly caused by physical movement, respiration, peristalsis, and vascular pulsation. Static anatomy produces a consistent signal, whereas moving components do not. Increasing the number of acquisitions will improve the chance of acquiring signals from static objects, reducing motion artefacts in the resultant image. However, this method itself will not completely remove motion artefacts and may result in longer scan times. More practical methods to counteract motion artefact include respiratory gating/triggers, respiratory compensation (phase re-ordering), radial k-space filling (BLADE or PROPELLER), and navigator echoes
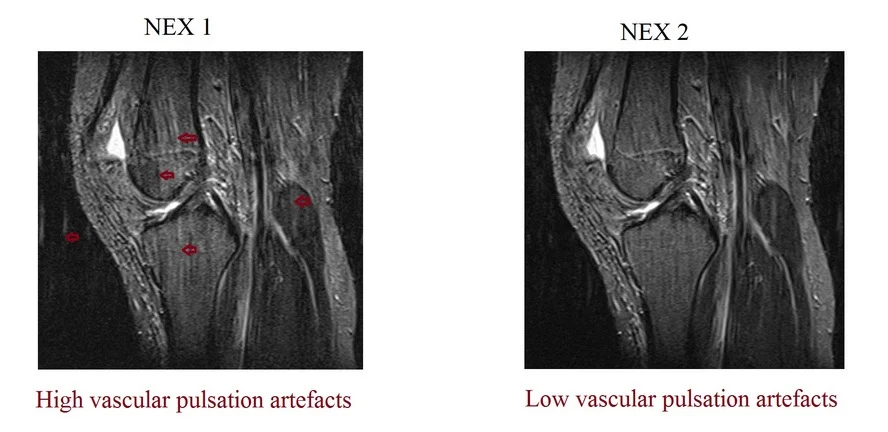
References
Bushberg, J. T., Seibert, J. A., Leidholdt, E. M., & Boone, J. M. (Eds.). (2011). The Essential Physics of Medical Imaging (3rd ed.). Lippincott Williams & Wilkins.
Haacke, E. M., Brown, R. W., Thompson, M. R., & Venkatesan, R. (2017). Magnetic Resonance Imaging: Physical Principles and Sequence Design (2nd ed.). Wiley.
Westbrook, C., & Roth, C. (2011). The Physics of MRI. Medical Physics Publishing.
Haacke, E. M., Brown, R. W., Thompson, M. R., & Venkatesan, R. (1999). Magnetic Resonance Imaging: Physical Principles and Sequence Design. John Wiley & Sons.
Bushberg, J. T., Seibert, J. A., Leidholdt Jr, E. M., & Boone, J. M. (2011). The Essential Physics of Medical Imaging. Lippincott Williams & Wilkins.
Callaghan, P. T. (1991). Principles of nuclear magnetic resonance microscopy. Clarendon Press.
Edelman, R. R., & Warach, S. (1993). Clinical applications of MR angiography. American Journal of Neuroradiology, 14(3), 607-612.
Jackson, E. F. (2017). MRI at a Glance. John Wiley & Sons.